The Next Generation IT Security System
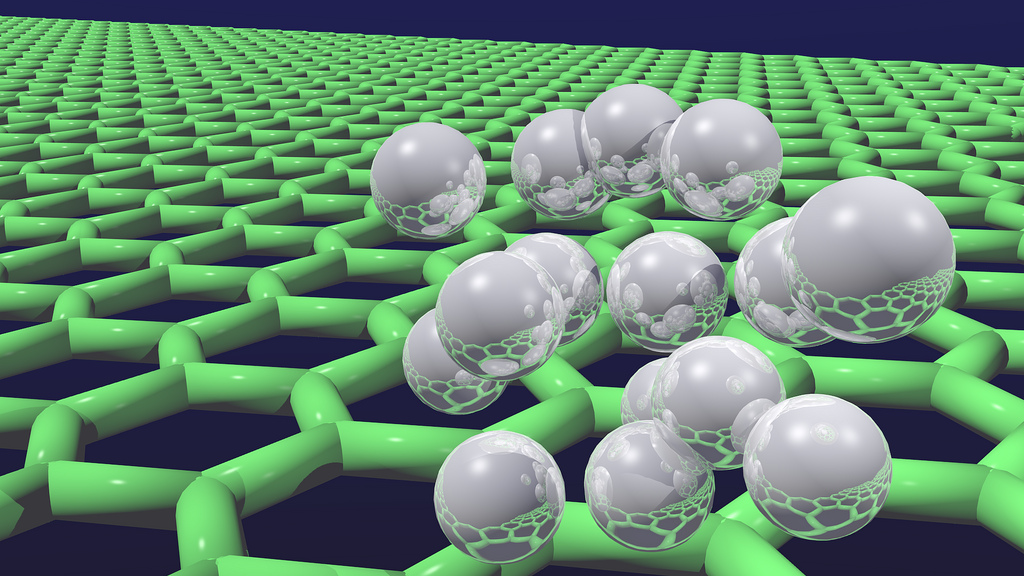
Spies, communication, and secret codes! Cryptography is the art of encrypting and decrypting messages. It has existed as long as people have distrusted each other and looked for the forms of secure communication. Cryptographic techniques have evolved over the centuries. The aim is always the same – the code-makers work to stay ahead of the code-breakers. Today’s most common encryption methods are threatened by the potential creation of the quantum computer. In other words, quantum cryptography promises more secure communication than any existing technique.
Introduction
Quantum cryptography is focused on the unique behavior of microscopic objects which enable users to securely develop secret keys. The work on quantum cryptography was begun by Stephen J. Wiesner in the late 1960’s. The first protocol for sending a private key using quantum techniques is published in 1984 by Bennett and Brassard. The development of quantum cryptography is based on “public-key” or “secret-key” methods.
Public-key encryption is related to the idea of a safe with two keys: a public key to lock the safe and a private key to open it. Using this method, anyone can send a message while the public key is used to encrypt messages, but only someone with the private key can decrypt the messages. The encrypting and decrypting keys are different.
On the other hand, secret-key encryption requires that two users first develop and securely share a secret key, which is a long string of randomly-chosen bits. The users then use the secret key along with public algorithms to encrypt and decrypt messages. The algorithms are very complex.
Quantum cryptography solves the problems of secret-key cryptography by providing a way for two users who are in different locations to securely establish a secret key and to detect if eavesdropping has occurred. Quantum cryptography accomplishes these remarkable feats by exploiting the properties of microscopic objects such as photons.
Photon Properties
Photons are some quite amazing particles. In general, they have no mass, they’re the smallest measure of light, and they can exist in all of their possible states at once, called the wave function. Light in this state is called unpolarized. This is exactly the same as if you constantly moved left, right, forward, backward, and up-and-down at the same time. Quite tricky, is not it? Yes! Do not be discouraged – even quantum physicists are struggling with the implications of the wave function.
The foundation of quantum physics is the unpredictability factor. This unpredictability is pretty much defined by Heisenberg’s Uncertainty Principle. By this principle, it is impossible to know both an object’s position and velocity – at the same time.
When dealing with photons for encryption, Heisenberg’s principle can be used as an advantage. In order to create a photon, quantum cryptographers use LEDs – light emitting diodes which is a source of unpolarized light. Through the use of polarization filters, we can force the photon to take one state or another. In other words, we can polarize it.
The thing about photons is that once they are polarized, they cannot be accurately measured again, except by a filter like the one that initially produced their current spin. So if a photon with a vertical spin is measured through a diagonal filter, either the photon will not pass through the filter or the filter will affect the photon’s behavior, causing it to take a diagonal spin. In this sense, the information on the photon’s original polarization is lost.
An Example of Quantum Cryptology Application
Quantum cryptography uses photons to transmit a key. Once the key is transmitted, coding and encoding using the normal secret-key method can take place. But how does a photon become a key? How do you attach information to a photon’s spin?
We will explain this using our well-known characters – Alice and Bob. Alice can send her photons through randomly chosen filters and record the polarization of each photon. She will then know what photon polarizations Bob should receive. When Alice sends Bob her photons using an LED, she will randomly polarize them through either the X or the + filters. It means that each polarized photon has one of four possible states: (|), (–), (/) or ( ). As Bob receives these photons, he decides whether to measure each with either his + or X filter — he can’t use both filters together. Additionally, Bob has no idea what filter to use for each photon, he is guessing for each one.
After their conversation, Alice and Bob both throw out the results from Bob’s incorrect guesses. This leaves Alice and Bob with identical strings of polarized protons. To Alice and Bob, this is a meaningless string of photons. But once binary code is applied, the photons become a message.
Conclusion
Realization of practical quantum information technologies can not go forward without involvement of the network research community. The advances in computer processing power will remain a driving force in the continued research and development of quantum cryptography. The technology has the potential to make a valuable contribution to the network security among government, businesses, and academic sector.
References:
[1] Karen Hunter, Todd Duncan, Quantum Cryptography, SCI 510: QUANTUM, 2002.
[2] Vladimir L. Kurochkin,Igor G. Neizvestny, Quantum Cryptography, 10th International Conference and Seminar EDM 2009, 2009.
[3] Mehrdad S. Sharbaf, Quantum Cryptography: A New Generation of Information Technology Security System, 2009 Sixth International Conference on Information Technology: New Generations, 2009.
Djekic M (2014-01-14 00:15:59). The Next Generation IT Security System . Australian Science. Retrieved: Jul 12, 2025, from http://ozscience.com/technology/the-next-generation-it-security-system/