Life – Evolved
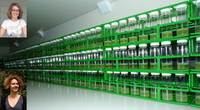
What is a life form? Most of us, if asked, will think of an animal or a human being-in other words, a multicellular organism. However, life itself evolved in cells, single cells of various types that existed and propagated independently. What are, then, the factors that led cells to collaborate together and give rise to complex organisms? Collaboration is possible only if there are no cheaters -cells that exploit the collaboration of others- and cheaters always arise. How is possible, then, for a multicellular organism to develop? Do the cheaters need to be eliminated –an evolutionary impossibility- for complex organisms to occur? This seems the classic common sense response. However, new and unexpected findings by a team of scientists working in New Zealand and the US, suggest the opposite: that the presence of cheaters can be instrumental to the formation of self-generating groups of cells, or collectives.
Evolution can be studied in many ways; fossil examination is a well-known example. However, for researchers looking to have results in a quicker fashion –and with the added bonus of controlling variables within their experiments- bacterial models are the gold standard. Bacteria are used as they grow fast, in most cases multiple generations in one day, and their visible characteristics (their “phenotype”) can be characterised in detail. For this work, the scientists used a bacterium called Pseudomonas fluorescens. Its peculiarity is that, when grown in a static test tube (ie, without any shaking), selection favours mutants that allow the formation of surface-colonising “mats”. The mutation triggers the production of a cell-cell glue; as a result, more cells clump together and, once anchored to the walls of the test tube, the mat stands to be sustained. This is an advantageous behaviour as cells can now use oxygen, which they would not have access to if they remained as single cells in the solution. But there is a “but”: in this setting, cheaters always arise. Cheaters here are cells that do not produce glue but still sit within the mat, thereby benefitting of the oxygen. If nothing selects against cheaters, they proliferate unchecked and the mat ultimately collapses. In this setting, cheaters appear to be detrimental-but what if that was not the case?
The researchers set up two different life cycles for the mats, each lasting 9 days (6 days the first phase, 3 days the second). They made sure that at the bottleneck between the two phases only one cell type, either mat-forming or cheater, made it through. In the first model, mats were allowed to reproduce starting from the cheaters’ population. In this case, some of the cheaters had to “switch” and become normal cells again to produce the glue, otherwise there would have been no mat in the end. Here, cheaters become a seed -a germ line, in biology jargon- to reproduce the entire system (ie the mat). In the second model, mats were propagated by fragmentation, cheaters were purged from the system, and normal cells were used as a seed. After the cycle, the viability of the mats improved- in both cases, as expected, evolution worked. But, counter-intuitively, in the first case the “fitness” (evolutionary success) of the single cells that built the mat was lower than the fitness of the mat as a whole. In biological jargon, the fitness of the collective decoupled from the fitness of the single cells. This is a crucial finding, because it is precisely what we would expect to be the first step to generate a true multicellular organism-some cells have to “give up” to allow the harmonious growth of something bigger and better than themselves. In this system, individual cells within the mats of the first type ended up working for the common good, relinquishing a part of their viability but contributing to the generation of a collective that is more than a casual cluster of cells.
We asked the lead author of the study, Prof Paul B. Rainey, about the biological problem he and his colleagues had set out to address. He replied: “We wanted to understand how groups of cells evolved the capacity for reproduction”. He then explained: “Single cells can divide, but this does not guarantee that groups of cells can divide (reproduce). Groups that cannot reproduce cannot evolve by natural selection. If they cannot evolve there is no possibility of them being a precursor for the evolution of multicellular life. Pondering this problem led to the idea that cheaters might be functionally integrated into a life cycle that would allow reproduction of groups via a “seed” (a germ line) derived from cheaters”.
Prof Rainey continued to explain the significance of the life cycle: “A life cycle establishes a time scale longer than that of the individual cell. This provides possibility for natural selection to act over this longer time scale. When this happens, selection favours groups that perpetuate the life cycle and eliminates groups that do not. In essence, selection over the longer time scale acts on a developmental programme (a property of the group) that transitions cells between group and single cell phases. While selection continues to act on the short-term interests of cells, rapid growth is unlikely to aid perpetuation of the life cycle. Ultimately, if selection on groups is successful, the interests of cells become aligned with those of the group, even though this means that individual cells will come to relinquish some of their own reproductive autonomy. This leads to a decoupling of fitness between groups and cells, as seen in our experiments.”
In conclusion, Prof Rainey comments: “We did not observe decoupling when we evolved groups via a life cycle that involved reproduction via fragmentation. This is because when reproduction occurs via fragmentation natural selection just sees “more of the same”. In contrast, the two phase life cycle involving cheaters and cooperating cells provide selection with an altogether new kind of biological individual.”
You can find the whole paper here:
http://www.nature.com/nature/journal/v515/n7525/full/nature13884.html
CAPTION FOR THE PICTURE:
Caroline Rose (top) and Katrin Hammerschmidt and a sample of the microcosms they used to evolve multicellular life