The (nuclear) alchemists of Darmstadt and the doubly magic tin-100 nucleus
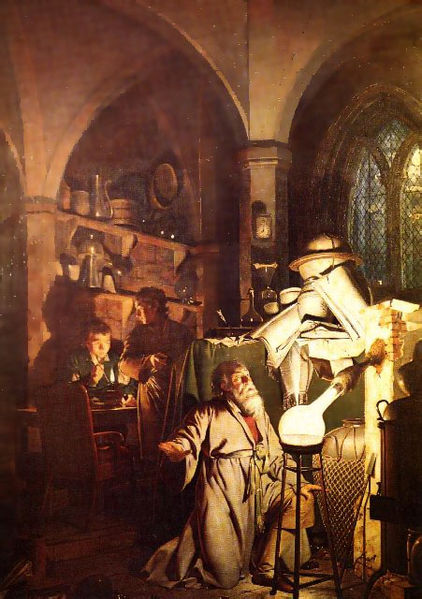
An international group of researchers announced in the journal Nature that they had succeeded in creating tin-100. This experiment helps us understand how heavy elements have formed. A few minutes after the Big Bang the universe contained no other elements than the lightest; hydrogen and helium.
We, the objects around us, the Earth and the other planets all contain heavier elements; carbon, oxygen, silicon, tin, iron etc. These elements came into existence later than hydrogen and helium. They formed through the fusion of atomic nuclei inside of stars. Elements heavier than iron owe their existence to gigantic stellar explosions called supernovas. Tin-100 is a very unstable, yet important, element for the understanding the formation of these heavier elements.
A multinational team headed by nuclear physicists from the Technische Universitat Munchen, the Cluster of Excellence Origin and Structures of the Universe and the GSI in Darmstadt carried out these precision experiments. They shot xenon-124 ions at a sheet of beryllium to create the tin-100 atoms. The subsequently measured the half-life and decay energy of tin-100 and its decay products using specially developed particle detectors.
What is our world made from?
The inspiration of creating new elements can be traced to alchemical traditions. Alchemy is an arcane tradition, that can be viewed as a proto-science, a precursor to chemistry and nuclear physics. It’s prime objective was to produce the mythical philosopher’s stone, which was said to be capable of turning base metals into gold or silver, and also act as an elixir of life that would confer youth and immortality upon its user.
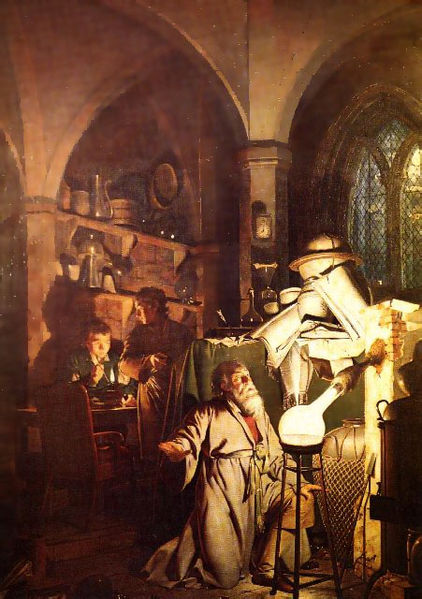
It did bring to chemistry many ideas and provided procedures, equipment, and terminology that are still in use. It also provided the inspiration for the creation of new elements. Now we understand to create new elements requires a combination of precision equipment and experimental procedures coupled with a sound understanding of quantum theory.
So what is tin-100 and why is it useful to understand the astrophysics of heavy element formation?
Most people will recognise that matter around us is composed of atoms. Atoms of carbon, hydrogen, oxygen for example form the building blocks to make organic molecules and silicon and oxygen bond together to make common beach sand and are fused together to make glass. The familiar metals are solids made of one type of atom, for example gold and aluminium, or combinations, bronze being made of copper and tin atoms.
Atoms in turn are a central nucleus of protons and neutrons surrounded by a swarm of electrons. The number of protons distinguishes one element from another. This atomic number is used to designate an element 1 for hydrogen, 8 for oxygen and 50 for tin, for example. Stable tin comprises 112 nuclear particles – 50 protons and 62 neutrons. The neutrons act as a kind of buffer between the electrically repelling protons and prevent normal tin from decaying. Each atom will contain an equal number of electrons to its protons. Remove or add an electron and the atom becomes an ion, a charged particle.
The strange quantum world of the nuclei
Quantum mechanics which, amongst other things, explains how the electrons form into shells around the nucleus. Elements which have filled outer shells, helium, neon, argon, xenon are ‘noble’ gases, chemically inert – not the least reactive. Nuclei are also complex quantum objects.
As far as we know, nuclei are the smallest objects that can be split up into their constituents. They are therefore the smallest entities which emergent properties – patterns that arise from complexity – can be studied. Nuclear scientists study these emergent phenomena and are using them to decipher the nature of the nuclear force. In contrast to the structure of atoms, for which the fundamental interaction between the electrons and the nucleus – the electromagnetic force – is known with great precision, the interaction between the nucleons – the strong nuclear force – is not so well known.
In nature not all combinations of nucleons are stable. As a general rule the more protons present then more neutrons are required to stablise the nuclei. A useful graphical presentation of this is the Segre table of radionuclides.
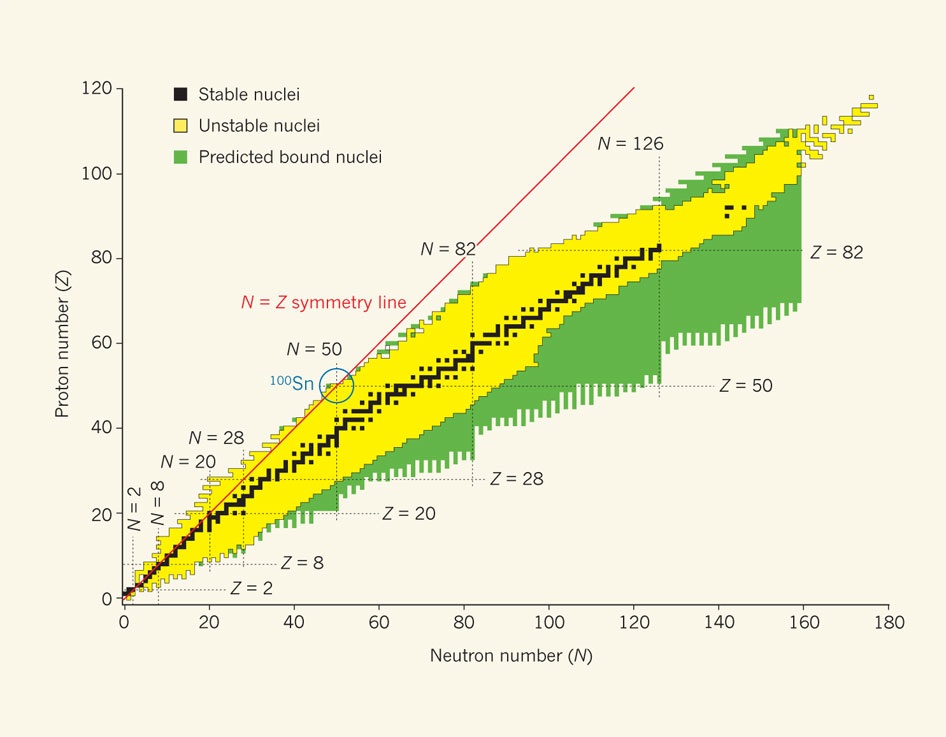
If the shell structure of electrons was difficult at first for scientists to come to terms with, then the shell structure exhibited by nucleons is not only unexpected it is complex enough not to be discussed in many quantum physics texts. It was first thought that such densely packed and strongly interacting objects as the nucleons would exhibit a liquid-like behavior, much like the flow of electrons in a good conductor such as a metal.
That is what makes these experiments so exciting.
Stability and magic numbers
Magic numbers are the number of protons or neutrons that form full shells in an atomic nucleus. The term is thought to have been coined by the physicist Eugene Wigner. The model has been used to explain – at least for stable nuclei – the observed sequence of magic numbers: 2, 8, 28, 50, 82 and 126.
Nuclei that have a magic number of neutrons or protons are more tightly bound than there non-magic counterparts. This intrinsic simplicity makes them prime candidates for testing proposed models of nuclear structure. Even more attractive are the doubly magic nuclei. The lighter nuclei helium-4, oxygen-16 and calcium-40 do follow the magic number sequence.
However because of the repulsion between protons the line of stable nuclei veers away from the symmetry line. As a result tin-100 represents the largest nuclei to follow the sequence. It is bound but unstable. It is very close to the edge of nuclear stability, where the nuclear force between the protons and neutrons can no longer bind them into a nucleus. Unfortunately, what makes this nucleus so attractive to study is what also makes it so difficult.
How to make a new element
In nature elements heavier than iron come into being only in powerful stellar explosions – supernovas. These include, for example, the precious metals gold and silver and the radioactive uranium. The cauldron of a supernova gives rise to a whole array of high-mass atomic nuclei. these decay to stable elements via different short-lived intermediate stages.
There are two ways to create new elements in the laboratory. The first is is to fuse two nuclei in a manner that minimises the loss of protons or α-particles (helium-4 nuclei). The second is is more brutal, fragmenting a small part off a heavier nuclei in a collision.
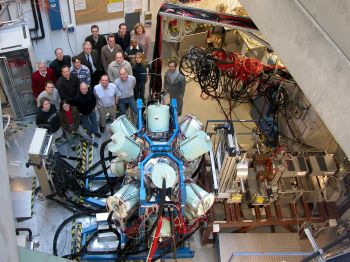
In these experiments energetic xenon-124 is sheared by making it collide with a target beryllium foil leaving a residue that is composed of 50 neutrons and 50 protons. Out of the 120,000,000,000,000 xenon-124 accelerated in the experiment, only 259 tin-100 nuclei were identified. These results were sufficient though for the decay of tin-100 to be studied with great precision.
The results, excitedly for the researchers, demonstrated a ‘superallowed Gamow-Teller decay‘. This type of β-decay is beyond the scope of this essay to explain, needless to say it does provide new experimental depth to the models of nuclear chemistry. It is an important decay transition that occurs in the collapse of supernovae. It also is important in putting boundaries on the possible mass of the neutrino. Both of which are important validations of the current nuclear theories as well as providing real experimental data to fine tune the theoretical models.
This allows more real models of nuclear synthesis to be constructed. Allowing a deeper understanding of how the atoms that make up our universe were created.
Now other laboratories around the world will work on improving the production rates of tin-100 and other exotic nuclei, based on these experiments. Allowing the emergent properties of these nuclei can be studied in more detail. Giving us greater understanding of the forces that bind these particles together – to make us!
1 thought on “The (nuclear) alchemists of Darmstadt and the doubly magic tin-100 nucleus”
Comments are closed.